- Mg+2 Valence Electrons
- Periodic Table With Valence Electrons Labeled
- Mg Element Valence Electrons
- Mg Has How Many Valence Electrons
- Mg Element Valence Electrons
- Mg+2 Valence Electrons
- Mg Valence Electrons
- Magnesium and fluorine combine to form an ionic compound. What is the formula for the compound? Mg most commonly forms a 2+ ion. This is because Mg has two valence electrons and it would like to get rid of those two ions to obey the octet rule.
- Answered 3 years ago Author has 79 answers and 210.5K answer views The atomic number of magnesium is 12. Therefore, the last filled orbital is the 3s orbital with two electrons pairing up. Therefore, n = 3 (principal energy level for 3s) and l = 0 (for s orbital).
The electrons in the 4d55s1 constitute its valence electrons. The symbol for molybdenum is Mo. It is classified as a transition metal and placed in group 6 or d-block and period 5 of the periodic table of elements. Valence electrons, their variation in the periodic table and relation to reactivity and electrical conductivity of elements.
Introduction
This section continues to explore the relationship between an atom’s electron arrangement in orbitals and the chemical properties of that atom. As we move from hydrogen to multi-electron atoms there is an incredible increase in complexity due to the fact that electrons repel each other. Nonetheless, one can still understand much of the periodic table and the trends in properties of atoms and ions by using approximations that are based on using the quantum numbers of individual electrons in an atom. These properties vary periodically as the electronic structure of the elements changes. They are (1) size (radius) of atoms and ions, (2) ionization energies, and (3) electron affinities. This section will focus on the properties governing the size (radius) of atoms and ions.
Learning Objectives for Core and Valence Electrons, Shielding, Zeff
- Correlate the effective nuclear charge with selected trends in periodic properties.
| Effective Nuclear Charge (Zeff) | Shielding | Variation in Atomic Radius |
| Key Concepts and Summary | Key Equations | Glossary |End of Section Exercises |
Effective Nuclear Charge (Zeff)
For an atom or an ion with only a single electron, we can calculate the potential energy of an electron by considering only the electrostatic attraction between the positively charged nucleus and the negatively charged electron. When more than one electron is present, however, the total energy of the atom or the ion depends not only on attractive electron-nucleus interactions but also on repulsive electron-electron interactions. For example, in helium there are two electrons. From Coloumb’s law we know that there is a repulsive interaction that depends on the distance between them. In addition there are attractive interactions between each of the two electrons with the nucleus. There are no known solutions to the Schrodinger equation for this problem, so one must use approximate methods to find the orbitals and their energies.
Mac mini memory upgrade 2020. If an electron is far from the nucleus (i.e., if the distance r between the nucleus and the electron is large), then at any given moment, most of the other electrons will be between that electron and the nucleus. Hence these electrons will cancel a portion of the positive charge of the nucleus and thereby decrease the attractive interaction between the nucleus and the electron farther away. As a result, the electron farther away experiences an effective nuclear charge (Zeff). An effective nuclear charge is the nuclear charge an electron actually experiences because of shielding from other electrons closer to the nucleus (Figure 1). Consequently, the Zeff is always less than the actual nuclear charge, Z. The Zeff experienced by an electron in a given orbital depends not only on the spatial distribution of the electron in that orbital but also on the distribution of all the other electrons present.
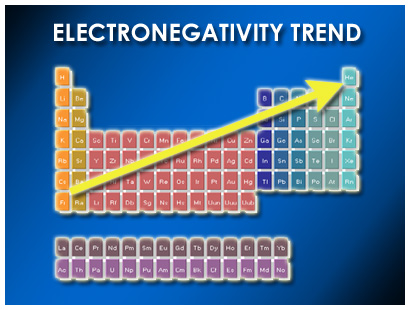
Note that while we often refer to the Zeff of a valence electron, we can calculate the Zeff for any electron by taking into account only the number of core electrons that are shielding. For example, consider a 2s electron of Cl. For Cl, Z = 17 and the electron configuration is 1s22s22p63s23p5. The only electrons that will shield a 2s electron are the 1s electrons, and there are two of them. Therefore, Zeff = 17 – 2 = 15 for a 2s electron of Cl. The Zeff for a 3p electron, on the other hand, is Zeff = 17 – 10 = 7 because there are 10 electrons shielding the 3p electron (2 electrons in n = 1 and a total of 8 electrons in n = 2).
Variation in Atomic Radius
The quantum mechanical picture makes it difficult to establish a definite size of an atom. However, there are several practical ways to define the radius of atoms and, thus, to determine their relative sizes that give roughly similar values. We will use the atomic radii (Figure 2), which is defined as one-half the distance between the nuclei of two identical atoms when they are joined by a covalent bond (this measurement is possible because atoms within molecules still retain much of their atomic identity). We know that as we scan down a group, the principal quantum number, n, increases by one for each element. Thus, the electrons are being added to a region of space that is increasingly distant from the nucleus. Consequently, the size of the atom (and its atomic radius) must increase as we increase the distance of the outermost electrons from the nucleus. This trend is illustrated for the atomic radii of the halogens in Table 1 and Figure 2. The trends for the entire periodic table can be seen in Figure 2.
Atom | Atomic radius (pm) | Nuclear charge |
---|---|---|
F | 64 | +9 |
Cl | 99 | +17 |
Br | 114 | +35 |
I | 133 | +53 |
At | 148 | +85 |
Table 1. Covalent Radii of the Halogen Group Elements |
As shown in Figure 2, as we move across a period from left to right, we generally find that each element has a smaller atomic radius than the element preceding it. This is expected due to the Zeff for the valence electron increasing from left to right across a period, as we saw above. The stronger pull (higher effective nuclear charge) experienced by valence electrons on the right side of the periodic table draws them closer to the nucleus, making the atomic radii smaller.
Explore visualizations of the periodic trends discussed in this section (and many more trends). With just a few clicks, you can create three-dimensional versions of the periodic table showing atomic size or graphs of ionization energies from all measured elements.
Example 1
Sorting Atomic Radii
Predict the order of increasing atomic radius for Ge, Fl, Br, Kr.
Solution
Radius increases as we move down a group, so Ge < Fl (Note: Fl is the symbol for flerovium, element 114, NOT fluorine). Radius decreases as we move across a period, so Kr < Br < Ge. Putting the trends together, we obtain Kr < Br < Ge < Fl.
Check Your Learning
Give an example of an atom whose size is smaller than fluorine.
Key Concepts and Summary
Effective nuclear charge for valence electrons increases from left to right across a period and decreases down a group. Because valence electrons are held more tightly on the right side of the Periodic Table, the atomic radius decreases. Atomic radius increases as we move down a group because the n level (orbital size) increases.
Key Equations
- Zeff = Z – S, where S is the number of core electrons
Glossary
- atomic radius
- one-half the distance between the nuclei of two identical atoms when they are joined by a covalent bond
- core electrons
- electrons occupying the inner shell orbitals
- effective nuclear charge
- charge that leads to the Coulomb force exerted by the nucleus on an electron, calculated as the nuclear charge minus shielding
- valence electrons
- electrons in the outermost or valence shell (highest value of n) of a ground-state atom; determine how an element reacts
- Based on their positions in the periodic table, predict which has the smallest atomic radius: Mg, Sr, Si, Cl, I.
- Based on their positions in the periodic table, predict which has the largest atomic radius: Li, Rb, N, F, I.
- Atoms of which group in the periodic table have a valence shell electron configuration of ns2np3?
- Atoms of which group in the periodic table have a valence shell electron configuration of ns2?
- Based on their positions in the periodic table, list the following atoms in order of increasing radius: Mg, Ca, Rb, Cs.
- Based on their positions in the periodic table, list the following atoms in order of increasing radius: Sr, Ca, Si, Cl.
Answers to Chemistry End of Section Exercises
- Cl
- Rb
- 15 (5A)
- 2 (2A)
- Mg < Ca < Rb < Cs
- Cl < Si < Ca < Sr
Introduction to Bonding
Chemical bonding describes a variety of interactions that hold atoms together in chemical compounds.
Learning Objectives
List the types of chemical bonds and their general properties
Key Takeaways
Key Points
- Chemical bonds are forces that hold atoms together to make compounds or molecules.
- Chemical bonds include covalent, polar covalent, and ionic bonds.
- Atoms with relatively similar electronegativities share electrons between them and are connected by covalent bonds.
- Atoms with large differences in electronegativity transfer electrons to form ions. The ions then are attracted to each other. This attraction is known as an ionic bond.
Key Terms
- bond: A link or force between neighboring atoms in a molecule or compound.
- ionic bond: An attraction between two ions used to create an ionic compound. This attraction usually forms between a metal and a non-metal.
- covalent bond: An interaction between two atoms, which involves the sharing of one or more electrons to help each atom satisfy the octet rule. This interaction typically forms between two non-metals.
- intramolecular: Refers to interactions within a molecule.
- intermolecular forces: Refers to interactions between two or more molecules.
Chemical bonds
Chemical bonds are the connections between atoms in a molecule. These bonds include both strong intramolecular interactions, such as covalent and ionic bonds. They are related to weaker intermolecular forces, such as dipole-dipole interactions, the London dispersion forces, and hydrogen bonding. The weaker forces will be discussed in a later concept.
Chemical bonds: This pictures shows examples of chemical bonding using Lewis dot notation. Hydrogen and carbon are not bonded, while in water there is a single bond between each hydrogen and oxygen. Bonds, especially covalent bonds, are often represented as lines between bonded atoms. Acetylene has a triple bond, a special type of covalent bond that will be discussed later.
Covalent Bonds
Chemical bonds are the forces of attraction that tie atoms together. Bonds are formed when valence electrons, the electrons in the outermost electronic “shell” of an atom, interact. The nature of the interaction between the atoms depends on their relative electronegativity. Atoms with equal or similar electronegativity form covalent bonds, in which the valence electron density is shared between the two atoms. The electron density resides between the atoms and is attracted to both nuclei. This type of bond forms most frequently between two non- metals.
When there is a greater electronegativity difference than between covalently bonded atoms, the pair of atoms usually forms a polar covalent bond. The electrons are still shared between the atoms, but the electrons are not equally attracted to both elements. As a result, the electrons tend to be found near one particular atom most of the time. Again, polar covalent bonds tend to occur between non-metals.
Ionic Bonds
Finally, for atoms with the largest electronegativity differences (such as metals bonding with nonmetals), the bonding interaction is called ionic, and the valence electrons are typically represented as being transferred from the metal atom to the nonmetal. Once the electrons have been transferred to the non-metal, both the metal and the non-metal are considered to be ions. The two oppositely charged ions attract each other to form an ionic compound.
Bonds, Stability, and Compounds
Covalent interactions are directional and depend on orbital overlap, while ionic interactions have no particular directionality. Each of these interactions allows the atoms involved to gain eight electrons in their valence shell, satisfying the octet rule and making the atoms more stable.
These atomic properties help describe the macroscopic properties of compounds. For example, smaller covalent compounds that are held together by weaker bonds are frequently soft and malleable. On the other hand, longer-range covalent interactions can be quite strong, making their compounds very durable. Ionic compounds, though composed of strong bonding interactions, tend to form brittle crystalline lattices.
Ionic Bonds
Ionic bonds are a subset of chemical bonds that result from the transfer of valence electrons, typically between a metal and a nonmetal.
Learning Objectives
Summarize the characteristic features of ionic bonds
Key Takeaways
Key Points
- Ionic bonds are formed through the exchange of valence electrons between atoms, typically a metal and a nonmetal.
- The loss or gain of valence electrons allows ions to obey the octet rule and become more stable.
- Ionic compounds are typically neutral. Therefore, ions combine in ways that neutralize their charges.
Key Terms
Mg+2 Valence Electrons
- valence electrons: The electrons of an atom that can participate in the formation of chemical bonds with other atoms. They are the furthest electrons from the nucleus.
- octet rule: An atom is most stable when there are eight electrons in its valence shell.
Forming an Ion
Ionic bonds are a class of chemical bonds that result from the exchange of one or more valence electrons from one atom, typically a metal, to another, typically a nonmetal. This electron exchange results in an electrostatic attraction between the two atoms called an ionic bond. An atom that loses one or more valence electrons to become a positively charged ion is known as a cation, while an atom that gains electrons and becomes negatively charged is known as an anion.
This exchange of valence electrons allows ions to achieve electron configurations that mimic those of the noble gases, satisfying the octet rule. The octet rule states that an atom is most stable when there are eight electrons in its valence shell. Atoms with less than eight electrons tend to satisfy the duet rule, having two electrons in their valence shell. By satisfying the duet rule or the octet rule, ions are more stable.
A cation is indicated by a positive superscript charge (+ something) to the right of the atom. An anion is indicated by a negative superscript charge (- something) to the right of the atom. For example, if a sodium atom loses one electron, it will have one more proton than electron, giving it an overall +1 charge. The chemical symbol for the sodium ion is Na+1 or just Na+. Similarly, if a chlorine atom gains an extra electron, it becomes the chloride ion, Cl–. Both ions form because the ion is more stable than the atom due to the octet rule.
Forming an Ionic Bond
Once the oppositely charged ions form, they are attracted by their positive and negative charges and form an ionic compound. Ionic bonds are also formed when there is a large electronegativity difference between two atoms. This difference causes an unequal sharing of electrons such that one atom completely loses one or more electrons and the other atom gains one or more electrons, such as in the creation of an ionic bond between a metal atom (sodium) and a nonmetal (fluorine).
Periodic Table With Valence Electrons Labeled
Formation of sodium fluoride: The transfer of electrons and subsequent attraction of oppositely charged ions.
Determining the Formula of an Ionic Compound
To determine the chemical formulas of ionic compounds, the following two conditions must be satisfied:
- Each ion must obey the octet rule for maximum stability.
- Ions will combine in a way that the overall ionic compound will be neutral. In other words, the charges of the ions must balance out.
Magnesium and fluorine combine to form an ionic compound. What is the formula for the compound?
Mg most commonly forms a 2+ ion. This is because Mg has two valence electrons and it would like to get rid of those two ions to obey the octet rule. Fluorine has seven valence electrons and usually forms the F – ion because it gains one electron to satisfy the octet rule. When Mg2+ and F – combine to form an ionic compound, their charges must cancel out. Therefore, one Mg2+ needs two F – Last update macbook pro. ions to neutralize the charge. The 2+ of the Mg is balanced by having two -1 charged ions. Therefore, the formula of the compound is MgF2. The subscript two indicates that there are two fluorines that are ionically bonded to magnesium.
On the macroscopic scale, ionic compounds form crystalline lattice structures that are characterized by high melting and boiling points and good electrical conductivity when melted or solubilized.
Example
Magnesium and fluorine combine to form an ionic compound. What is the formula for the compound?
Mg most commonly forms a 2+ ion. This is because Mg has two valence electrons and it would like to get rid of those two ions to obey the octet rule. Fluorine has seven valence electrons and as such, usually forms the F– ion because it gains one electron to satisfy the octet rule. When Mg2+ and F– combine to form an ionic compound, their charges must cancel out. Therefore, one Mg2+ needs two F– ions to balance. The 2+ of the Mg is balanced by having two -1 charged ions. Therefore, the formula of the compound is MgF2. The subscript two indicates that there are two fluorines that are ionically bonded to magnesium.
Covalent Bonds
Covalent bonding involves two atoms, typically nonmetals, sharing valence electrons.
Learning Objectives
Differentiate between covalent and ionic bonds
Key Takeaways
Key Points
- Covalent bonds involve two atoms, typically nonmetals, that share electron density to form strong bonding interactions.
- Covalent bonds include single, double, and triple bonds and are composed of sigma and pi bonding interactions where 2, 4, or 6 electrons are shared respectively.
- Covalent compounds typically have lower melting and boiling points than ionic compounds.
Key Terms
Mg Element Valence Electrons
- electronegativity: The tendency of an atom or molecule to attract electrons and thus form bonds.
- single bond: A type of covalent bond where only two electrons are shared between atoms.
Forming Covalent Bonds
Covalent bonds are a class of chemical bonds where valence electrons are shared between two atoms, typically two nonmetals. The formation of a covalent bond allows the nonmetals to obey the octet rule and thus become more stable. For example:
- A fluorine atom has seven valence electrons. If it shares one electron with a carbon atom (which has four valence electrons), the fluorine will have a full octet (its seven electrons plus the one it is sharing with carbon).
- Carbon will then have five valence electrons (its four and the one its sharing with fluorine). Covalently sharing two electrons is also known as a “single bond.” Carbon will have to form four single bonds with four different fluorine atoms to fill its octet. The result is CF4 or carbon tetrafluoride.
Covalent bonding requires a specific orientation between atoms in order to achieve the overlap between bonding orbitals. Covalent bonding interactions include sigma-bonding (σ) and pi-bonding (π). Sigma bonds are the strongest type of covalent interaction and are formed via the overlap of atomic orbitals along the orbital axis. The overlapped orbitals allow the shared electrons to move freely between atoms. Pi bonds are a weaker type of covalent interactions and result from the overlap of two lobes of the interacting atomic orbitals above and below the orbital axis.
Mg Has How Many Valence Electrons
Covalent bonds can be single, double, and triple bonds.
Mg Element Valence Electrons
- Single bonds occur when two electrons are shared and are composed of one sigma bond between the two atoms.
- Double bonds occur when four electrons are shared between the two atoms and consist of one sigma bond and one pi bond.
- Triple bonds occur when six electrons are shared between the two atoms and consist of one sigma bond and two pi bonds (see later concept for more info about pi and sigma bonds).
Mg+2 Valence Electrons
Ionic Compounds v. Molecular Compounds
Mg Valence Electrons
Unlike an ionic bond, a covalent bond is stronger between two atoms with similar electronegativity. For atoms with equal electronegativity, the bond between them will be a non- polar covalent interaction. In non-polar covalent bonds, the electrons are equally shared between the two atoms. For atoms with differing electronegativity, the bond will be a polar covalent interaction, where the electrons will not be shared equally.
Ionic solids are generally characterized by high melting and boiling points along with brittle, crystalline structures. Covalent compounds, on the other hand, have lower melting and boiling points. Unlike ionic compounds, they are often not soluble in water and do not conduct electricity when solubilized.
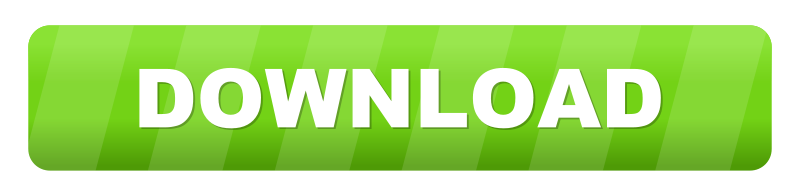